Edit: a few weeks after I wrote this post, a very interesting paper was published about experimentally inducing epigenetic inheritance in mice. I plan to write an update taking this into account.
The idea that environmental influences on parents can be inherited by their children is an old one, going back to at least Lamarck. In recent years, this idea has taken the form of “epigenetic inheritance”. For example, you may have seen studies claiming that if your grandma starved, you have a higher obesity risk,1 and that this has something to do with epigenetics.
However, this concept has been massively overhyped, especially in popular media. In this post, I will examine what “epigenetic inheritance” really means and review some studies about it in mammals.
Defining inheritance
Inheritance is defined as the transmission of variation from parents to offspring.
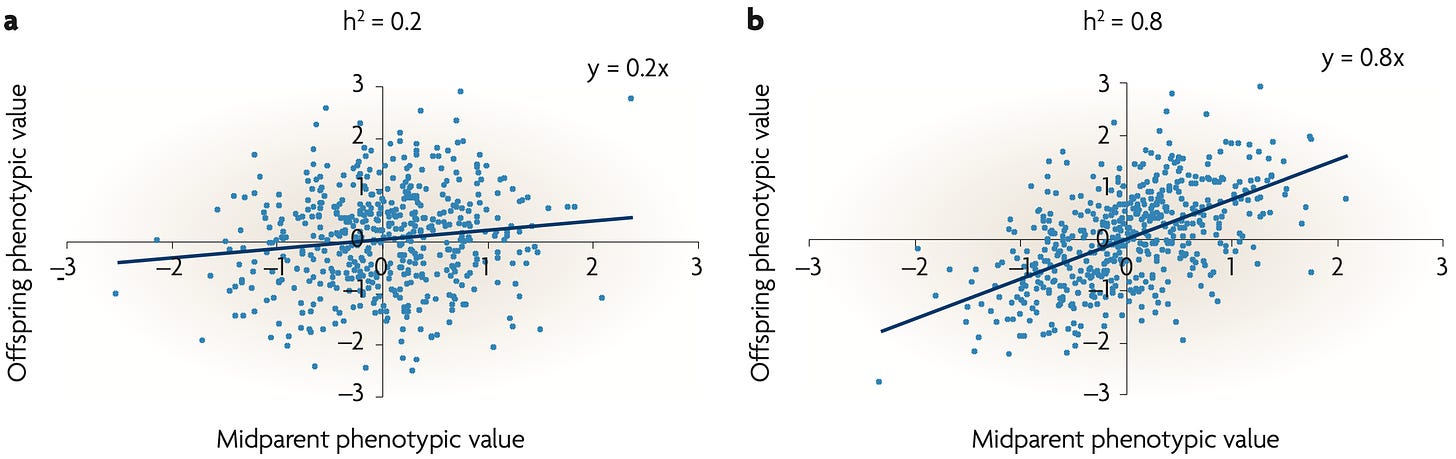
Let’s consider three sources of variation: genetic variation, epigenetic variation, and environmental variation. How do these interact?
Within a particular organism, there can be:
Genetic effects on environment. For example, mutations in immune-related genes alter the organism’s microbiome.
Genetic effects on epigenetic marks, within an organism. For example, mutations in a methyltransferase binding sequence can lead to different methylation levels.2
Epigenetic effects on environment. For example, epigenetic regulation of olfactory genes leads to eating different foods.
Environment effects on epigenetic marks. For example, folate deficiency means methyltransferase enzymes can’t function.
Note, in this diagram I am ignoring effects of epigenetic marks and environmental mutagens on genetic mutation rates. These effects exist,3 but are stochastic and don’t contribute to causal information flow.
Now consider the transmission of variation from parent (F0) to offspring (F1):
Offspring inherit genetic variation present in their parents.
Genetic variants present in the parent can affect epigenetic marks in the gametes, even if those genetic variants are not passed on to the offspring.4
Genetic variants present in the parent can affect the environment of the offspring, for example by altering parenting behavior.
Epigenetic variation present in the parent’s germline can be directly inherited by the offspring.
Similarly to #4, epigenetic variation in the parent can affect the environment of the offspring.
Environmental variation in the parents can affect the environment of the offspring. Examples include vertical transmission of microbes, or parenting behavior.
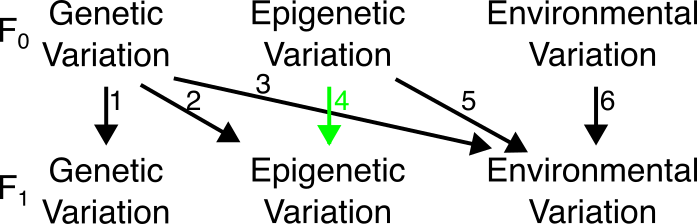
Epigenetic inheritance is when a variation in epigenetic marks is transmitted from a parent to their offspring. This corresponds to #4, and only #4. For the purposes of studying epigenetic inheritance, everything else is a confounder.
At this point, we should make a further distinction:
Intergenerational epigenetic inheritance is transmission of an epigenetic variation to the offspring of the organism that received the epigenetic variation. For example, if a man dips his balls in methyl iodide on a dare,5 later on, his kids might have some methyl groups where they shouldn’t. But those kids’ gametes will have likely erased the extra methyl groups, in which case the effect won’t be inherited in subsequent generations.
This can sometimes be confusing when the epigenetic perturbation happens to a fetus. For example, if your grandmother was malnourished while pregnant with your parent, then you might have some epigenetic problems. But this is still over one generation, since your parent was the one who received the epigenetic perturbation.
Transgenerational epigenetic inheritance is stable transmission of an epigenetic variation over multiple generations. If your grandmother was malnourished before she was pregnant with your parent, and both your parent and you have some epigenetic variation as a result, then this would be transgenerational epigenetic inheritance.
Whereas there is good evidence for intergenerational epigenetic inheritance in mammals, the evidence for transgenerational inheritance is much weaker. Its effect, if any, on human phenotypic variation is negligible.
Barriers to epigenetic inheritance
In the previous post of this series, I explained that there are two major epigenetic erasure events during mammalian germline development. First, epigenetic marks in the gametes are largely (though not fully) erased during early embryonic development. Thus, any kind of epigenetic inheritance must involve marks that are not erased during this period.
Second, epigenetic marks are much more thoroughly erased in primordial germ cells prior to the formation of gametes. This is not relevant for intergenerational epigenetic inheritance, since this erasure does not affect somatic cells. However, it is a strong barrier to transgenerational inheritance. Any such inheritance would need to be in regions of the genome that escape this erasure, which restricts this to a few transposable elements. This mode of inheritance would also require that the mammalian germline has multiple stable “local minima” of epigenetic trajectories, and it is not clear that this is true.
Alternatively, epigenetic perturbations in gonadal somatic cells could lead (via perturbed signaling pathways) to perturbations in germ cells. However, it would be highly unlikely that this could precisely re-establish the original epigenetic perturbations. More likely, this would result in a generalized epigenetic instability.6
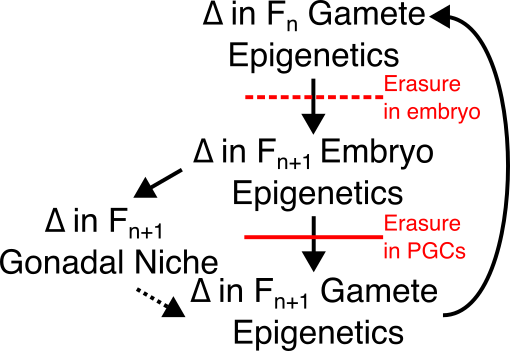
Reviewing the current evidence
Most studies of mammalian epigenetic inheritance are either rodent studies, or observational human studies. For each type, I will review one study that I consider to be notable and high-quality.
Mis-methylated mice
First, let’s consider a mouse model of transgenerational epigenetic inheritance (Blake et al. 2021). Here, the researchers studied mice with a mutation in the methyl-group metabolism gene Mttr. This mutation (Mttr^gt)7 broadly prevents DNA, RNA, and histone methylation. The wild-type offspring of mice heterozygous for the mutant allele (Mttr+/Mttr^gt) suffered from developmental problems, which consisted of a wide variety of abnormalities measured at 10.5 days of embryonic development. These effects could be established in the offspring of a mutant mouse of either sex, but were subsequently only transmitted through the female lineage. Some mice up to four generations removed from the mutant founder mice still had significant abnormalities in fetal development. Importantly, the effects still were observed when pregnancies were established in surrogate dams by IVF, thus ruling out environmental factors. The researchers also performed whole-genome sequencing on control and malformed embryos, showing that the malformed ones did not have an increased de novo mutation rate.8
To focus on the mechanism, the researchers measured genome-wide DNA methylation in the sperm of mutant and wild-type mice, and the embryos with and without developmental abnormalities. Interestingly, global methylation levels in the sperm did not significantly change due to the mutation. However, there were 203 regions at least 500 bp in size with significantly different methylation between sperm from wild-type and Mttr+/Mttr^gt mice. About half of these were associated with transposable elements. The researchers selected ten differentially methylated regions that were previously reported as escaping reprogramming in the early embryo, and measured their methylation in the embryos and placentas of the F1 and F2 generations, relative to normal mice. Notably, the differential DNA methylation from the sperm did not persist in the embryos and placentas, even when they showed developmental abnormalities.
At this point, the researchers still needed to figure out what was going on. To see if there were any transcriptional effects, they used RT-qPCR9 to measure mRNA expression levels from genes located near six of the ten differentially methylated regions. It’s not clear to me why they only chose six out of ten. (In my opinion, an unbiased look at the transcriptome using RNA-seq would have been much better.) One of the genes they investigated, Hira, had decreased mRNA expression in F2 embryos relative to normal controls, with this effect being stronger in severely defective embryos. Hira mRNA levels in placenta and liver were unaffected. Paradoxically, there was also increased Hira protein expression in the embryos, and decreased protein expression in the placentas (measured by Western blotting). This was only observed in F2, and not F1, embryos. Overall, this section of the paper is pretty weak, especially because most measurements were performed on only 3 – 5 embryos per group, and the methods (RT-qPCR and Western blotting) are prone to technical artifacts. It’s also not clear how Hira differential methylation in sperm can cause differences in Hira expression, given that the differential methylation does not persist.
In my view, this study is an upper bound on the strength of any transgenerational epigenetic inheritance. The researchers used a highly severe initial perturbation, and even in the F1 generation, the differential DNA methylation from the paternal sperm did not persist. The researchers suggest that other epigenetic effects may be responsible for the observed phenotypes, but do not provide evidence for this. Interestingly, the researchers compared their data to a previous study on the epigenetic effects of folate deficiency, which is like a milder form of Mttr inhibition. There were no differentially methylated regions shared between these two models. Overall, this study shows that a strong epigenetic perturbation can have developmental effects over multiple generations, but rules out the straightforward mechanism of persistent differential methylation, leaving the actual mechanism as an unanswered question.
What would a better mouse study look like?
Take two groups of genetically identical (inbred) mice. Expose one to an epigenetic perturbation and leave the other as a control.
Isolate gametes (eggs too, not just sperm) from each group. Measure epigenetic marks in the gametes,10 and also perform in vitro fertilization to generate embryos.
Transfer the embryos to surrogate mothers who were not exposed to the environmental perturbation.
Measure epigenetic marks in the F1 generation’s somatic cells and gametes. Be sure to measure the marks in the relevant cell types for the phenotype of interest.
If you’re trying to show transgenerational epigenetic inheritance, repeat the gamete isolation and IVF, and measure epigenetic marks again for the F2 generation (and possibly beyond).
Notably, epigenetic marks must be measured in the gametes for each generation, and any transmission of genetic or environmental variation must be excluded. Also, the experiment must have sufficient statistical power (i.e. use a large enough sample) to reliably detect the transmitted variation.
Hungry hungry humans
I was hoping to find a high-quality human study of transgenerational epigenetic inheritance, but despite spending a few hours on Pubmed, I couldn’t find a single one. If you find one, please post it in the comments and I’ll review it.
Instead, I will briefly discuss two studies, one of which is about epigenetic marks in adults who were prenatally undernourished, and the second about an increased risk of obesity in offspring of prenatally undernourished fathers. Both studies use the Dutch Hunger Winter Cohort, a group of people born in the Netherlands during and shortly after the winter of 1944–45. These people were prenatally undernourished because their mothers had their food supply cut off by the Nazis.11
The first study (Tobi et al. 2018) examines epigenetics but not inheritance. It found an increase in body mass index (+5.6%) and blood triglycerides (+13.5%) in these prenatally undernourished individuals later in life. Siblings born after the famine served as a control group. The researchers measured genome-wide DNA methylation in whole blood samples and identified one differentially methylated CpG site associated with prenatal famine exposure and BMI, and six sites associated with prenatal famine exposure and triglyceride levels. The New York Times published an article about this study, with the headline, “The Famine Ended 70 Years Ago, but Dutch Genes Still Bear Scars”.
Limitations of this study include:
Blood cells are not the most relevant cell type for studies on obesity. The authors try to justify their use of blood in the paper, but not to my satisfaction.
Whole blood contains multiple cell types with different epigenetic profiles, so observed epigenetic changes may actually reflect a shift in cell type abundances.
Increased adiposity may cause epigenetic changes, rather than the other way around.
They did not control for genetic effects (perhaps babies with a certain genotype were more likely to survive the famine, and also more likely to be obese).
Still, I think this is one of the better-quality studies about epigenetic effects of environmental disturbances on humans. Of course, it says nothing about inheritance.
The second study (Veenendall et al. 2013) interviewed the children of prenatally undernourished Dutch Hunger Winter survivors. To quote from the abstract,12
Adult offspring (F2) of prenatally exposed F1 fathers had higher weights and BMIs than offspring of prenatally unexposed F1 fathers (+4.9 kg, P = 0.03; +1.6 kg/m², P = 0.006). No such effect was found for the F2 offspring of prenatally exposed F1 mothers. We observed no differences in adult health between the F2 generation groups.
This study did observe an intergenerational effect, but it did not examine epigenetics in any way. As far as I know, no studies have yet done this. Nonetheless, in the discussion section of the study, the authors implied that epigenetics could be important. They also pointed out that they didn’t control for potential variations in parenting behavior or other environmental effects, so these also might explain the results. Of course, this story made its way into some rather hilarious school educational materials that only mentioned an epigenetic explanation.
What would a better human study look like?
Of course, running a randomized controlled trial of the Dutch Hunger Winter would make you the moral equivalent of the Nazis. However, the current observational studies could definitely be improved. Studies investigating obesity should take biopsies of adipose tissue in the F1 and F2 generations and measure the epigenetics. Sperm from F1 males would also be valuable to study (unfortunately, oocyte retrieval from F1 females is not feasible). Once epigenome-editing technology is better developed, it would also be interesting to reverse the differential methylation and see if this causes weight loss.
Common flaws in epigenetic inheritance studies
The studies I reviewed above were relatively decent compared to the vast majority of similar studies, which are crap. Here are some common problems to watch out for:
Not actually measuring epigenetic marks
Measuring epigenetic marks in irrelevant cell types that happen to be easy to sample13
Not controlling for the effects of genetic variation
Not preventing the transmission of environmental variation between generations14
Inadequate sample sizes
Not actually looking at inheritance
Not adequately adjusting for multiple comparisons (especially a problem in testing sex-specific effects)
Using non-mammalian organisms and generalizing to mammals (more of a problem in press releases and popular articles, rather than actual papers)
Reasoning about mechanisms and effects
As discussed above, there is some limited evidence for epigenetic inheritance in mammals, in cases of severe nutrient deprivation. How likely is this to be important?
For epigenetic inheritance to take place, an environmental perturbation first must be passed to the gametes and alter their epigenetic marks. The gonads are quite well-isolated from the rest of the body, so I find it implausible for something like olfactory exposure or psychologically traumatic stress to result in epigenetic inheritance. However, severe nutrient deprivation, especially during fetal development, could plausibly cause epigenetic effects in the gametes. Still, effects observed in humans were rather modest even when the parents were prenatally exposed to a severe famine.
Second, the altered marks in the gametes must cause a phenotypic change in the offspring. Although the epigenome is partially erased during embryonic development, the erasure might not be complete, or the altered marks could cause some other persistent phenotypic change before being erased (as happened in the mouse study I reviewed). However, transgenerational epigenetic inheritance can only happen when the variation in epigenetic marks escapes resetting in PGCs, and this is exceedingly unlikely in mammals. As I mentioned in the last post, I know of only one example, involving a differentially methylated transposable element in mutant mice.15
Overall, the epigenetic erasure between generations, as well as the relatively small magnitudes of effects in extreme, “upper-bound” studies such as the Dutch Hunger Winter Cohort, makes me think that epigenetic inheritance is much less important than genetic and environmental inheritance for explaining human phenotypic variation.
So why is the concept of epigenetic inheritance so popular?
I’m not sure. My best guess is that it provides a politically expedient explanation for the fact that certain historically traumatized groups are still disadvantaged today. The idea of “epigenetic trauma” neither implies that these groups are genetically inferior, nor that their culture or behavior is culpable for poor outcomes. Conveniently, it also absolves current society from responsibility by blaming the abuses of the past.
In any case, it seems that, just like the epigenome, this idea keeps being restored at each new generation. However, as a person with some great-grandparents who lived through famine and hardship, I’m personally glad that the popular conception of epigenetic inheritance is wrong.
Further reading
You can’t take it with you: straight talk about epigenetics and intergenerational trauma
A critical view on transgenerational epigenetic inheritance in humans
These are known as “secondary epimutations”. For one example see this study.
Notably, methylated cytosines are more likely to mutate to thymines.
For example, this study of effects of Y-chromosomal variation on daughter phenotypes in mice. But a larger study investigating different chromosomes didn’t find anything similar. Still, such effects are theoretically possible.
(These examples were helpfully provided by Mike Saint-Antoine here.)
Please don’t do this.
As seen below in the mouse study which I review.
Unfortunately Substack doesn’t allow superscripts, so I’m writing it like this.
This is important to check, because the Mttr mutation also interferes with DNA synthesis.
The same technology as used for SARS-CoV-2 tests. For an overview of practical considerations in making it reliable, as well as ways it can go wrong, see: https://www.bio-rad.com/webroot/web/pdf/lsr/literature/Bulletin_5859.pdf
In particular, these researchers measured the ratio of target mRNA to Gapdh mRNA. If Gapdh expression varied between samples (it is usually pretty stable but not always!), this would bias their results. As far as I know they didn’t check this.
It’s important to measure eggs and sperm separately since the epigenetic effects might be different in different kinds of gametes.
Food availability was <900 kcal/day for a period of several months.
They are defining F0 as the starving mothers, F1 as the starving fetuses, and F2 as their children. I would call the fetuses F0 since they are getting the environmental perturbations, but to keep things consistent, I am using the authors’ numbering.
For example, if you’re trying to show epigenetic inheritance of mental illness, you need to measure marks in brain tissue. If you’re trying to show transgenerational inheritance of any sort, you need to measure marks in gametes. However, most studies use blood or saliva.
This is hard to do for humans, but studies using adopted children can partially get around it.
As someone who works in epigenetics and has colleagues working on transgenerational inheritance, this article gets some stuff right, but it's also obvious that there are large gaps in the author's reading of the literature, which biases the interpretation of the field. I'd be happy to get specific if there's interest but I thought the larger readership should caveat emptor