Herpesvirus Treatment and Prevention
Part 7 of “The human herpesviruses: much more than you wanted to know”
This post brought to you by Amtrak, who cancelled my trip back to Boston, leaving me with more time to blog.
As we’ve seen in this series so far, herpesviruses, particularly CMV and EBV, cause a large burden of disease. Treating, or better yet, preventing, herpesvirus infections should therefore be a high priority for medical research.
Here I will discuss various approaches, and give an overview of existing therapies as well as opportunities for future ones.
1. Antiviral drugs
Several antiviral drugs have been developed, or are in development, to treat herpesvirus infections. I’ll discuss them grouped by their mechanism of action.
Nucleoside mimics
The basic idea of a nucleoside mimic is to exploit the virus’s need for rapid DNA synthesis. Viral nucleoside kinases (which phosphorylate nucleosides, allowing them to be polymerized into DNA) and polymerases (which do the polymerization) have sacrificed selectivity for additional speed.
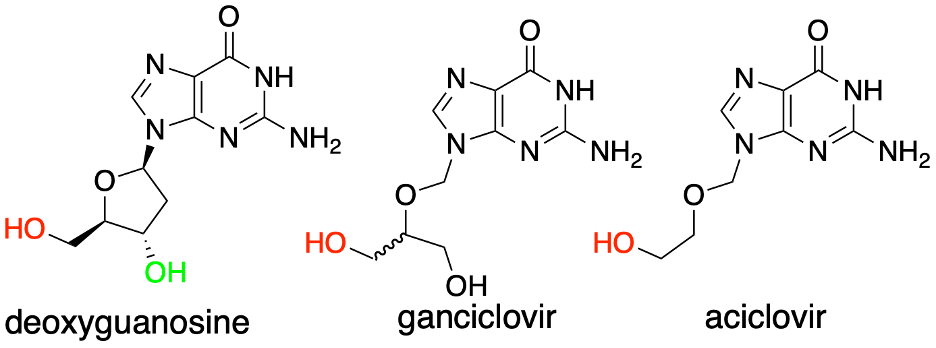
The deoxyguanosine mimics aciclovir, penciclovir, and ganciclovir all look roughly like deoxyguanosine (Figure 1), and are incorporated into DNA following phosphorylation by viral kinases. However, they lack the deoxyribose 3’-hydroxyl group that is necessary for further extension of the DNA, so as soon as they are incorporated, DNA synthesis stops. Due to differences in viral kinases, acyclovir and penciclovir work best against HSV and VZV (1, 2), while ganciclovir works best against CMV.
Prodrug versions of these (valaciclovir, valganciclovir, and famiciclovir) also exist. These are esters of the active drugs. The ester group (valyl or acetyl) helps with absorption when taken orally, and is cleaved by host esterases to release the active compound (Figure 2).
Importantly, the viral kinase is what drives toxicity. The only reason these drugs don’t kill healthy cells is that the human cells don’t phosphorylate them. Still, human nucleoside kinases aren’t perfectly selective. Thus, the drugs are also incorporated at lower levels into DNA of healthy host cells, which causes side effects. This is particularly problematic in pregnant women. CMV causes birth defects, but so does ganciclovir, which renders it useless in this patient population.
Besides deoxyguanosine mimics, there are also mimics for the other nucleosides, but these are even more toxic and mainly of historical interest. Vidarabine is an adenosine mimic. It’s basically adenosine but with arabinose instead of ribose, so the sugar has the wrong stereochemistry. Idoxuridine and trifluridine are thymidine mimics. Trifluridine has found use as an anti-cancer chemotherapy because it kills rapidly dividing cells. Most deoxycytidine mimics are even more toxic, and are only used for anti-cancer chemotherapy. One exception is cidofovir, which despite toxicity, is sometimes used to treat CMV infections. Strictly speaking, cidofovir is not a nucleoside mimic; since it’s already phosphorylated, it’s a nucleotide mimic. Interestingly, cidofovir has activity against smallpox, and its prodrug brincidofovir was approved in 2021 for this purpose.
The recently approved drug maribavir is somewhat similar to a nucleoside mimic, but instead of being phosphorylated by a viral kinase and incorporated into DNA, it inhibits the viral kinase. It was originally developed in the early 2000s, but failed to show efficacy in trials (3). However, it was approved in November 2021 for patients who did not respond to first-line anti-CMV treatments due to viral resistance.
Terminase inhibitors
Herpesviral replication proceeds by a rolling-circle mechanism, which generates a long concatemer of viral genomes. This concatemer must be cleaved to separate the viral genomes before they are packaged into mature virions. This cleavage is accomplished by the viral terminase enzyme, which is a target for inhibitors.
Letermovir is the first, and so far only, FDA-approved terminase inhibitor. It was approved in 2017 for CMV treatment and prophylaxis in immunosuppressed patients. Due to having fewer side effects than ganciclovir, it performs better in the prophylactic role (4). Letermovir is only effective against CMV, not other herpesviruses. It’s too new for generic versions to exist, so for now it’s about 10 times as expensive as ganciclovir.
Other potential targets for inhibition
Latency-associated proteins are particularly promising as targets, because inhibiting or degrading them has the potential to completely eliminate the virus. One paper reported developing EBNA1 inhibitors with the goal of treating EBV-driven cancers. The top molecule, called VK-1850, potently inhibits EBNA1 binding to DNA, and in a mouse model was successful at stopping nasopharyngeal carcinoma growth (5).
Unfortunately, a phase 1/2a trial of the subsequently developed compound VK2019 for EBV-positive nasopharyngeal carcinoma was terminated due to lack of efficacy. Although I don’t think the drug has been tested against EBV infection, to me it still seems like a very promising candidate.
This approach of targeting viral proteins necessary for latency would work best against EBV, CMV, and KSHV. HSV and VZV only express RNAs (not proteins) during latency, so these viruses don’t present useful targets for inhibition. HHV6 and HHV7 integrate into the host genome, so inhibiting viral proteins may not be effective at removing the virus.
2. Biologics
An antisense RNA therapy, fomivirsen, was developed to treat CMV retinitis. In general antisense RNAs suffer from poor delivery relative to small-molecule drugs, but for this case it can be injected directly into the eye, which results in direct delivery to the target tissue. However, fomivirsen was discontinued due to low demand after improved HIV treatments decreased CMV retinitis cases.
Various monoclonal antibodies have also been developed against herpesviruses, starting as early as the 1980s. However, in most herpesvirus infections the host’s immune system is perfectly good at making antibodies, it’s just that antibodies in general aren’t very effective against herpesviruses. Monoclonal antibodies have been suggested for immunosuppressed patients and premature infants, but even here efficacy has been lackluster (6, 7). Polyclonal antibody therapy, based on pooled serum antibodies from CMV-positive people, is FDA-approved but has low efficacy (7). A monoclonal antibody, rituximab, that eliminates B cells has been effective in treating EBV-related disease (8), but this comes with severe side effects, due to not having any B cells. Still, development of monoclonal antibodies continues; finding a better epitope for EBV neutralization has shown promise recently in a humanized mouse model (9).
3. Vaccines
Over the years, there have been many attempts at making human herpesviruses vaccines, with the only clear successes being against VZV. In general, vaccines are only as good as the human immune system, and because herpesviruses (especially EBV and CMV) are excellent at evading the immune system, vaccine development is challenging.
A key concept in anti-herpesvirus vaccine development is the distinction between a perfect vaccine, that prevents herpesvirus infection, and a good vaccine, that merely prevents symptoms. This is best illustrated by the example of Marek’s disease in chickens. Chickens can be vaccinated against the alphaherpesvirus that causes Marek’s disease, but this only prevents the disease, and the chickens can still be infected and shed the virus. Unfortunately, this has resulted in the evolution of more virulent strains that quickly kill unvaccinated chickens (10).
HSV Vaccines
Conceivably, a vaccine against HSV could both prevent infection, and also be useful for already infected patients to help prevent recurring outbreaks, just as a vaccine against VZV prevents shingles. Several approaches have been tested for making HSV vaccines, including live-attenuated viruses and a variety of protein-based vaccines (11). Unfortunately, no vaccine has yet shown acceptable efficacy in humans.
To date, there have only been three vaccine candidates that progressed to phase III trials, all of which were protein subunit vaccines (12–14). The most recent one was GlaxoSmithKline’s Herpevac, based on HSV2 glycoprotein D (14). Despite generating high levels of anti-gD antibodies in participants initially, by 16 months the median levels were undetectable. The vaccine showed no efficacy at all in men, and in women, had a moderate (estimated 35%) efficacy against HSV1 but not HSV2. Several other vaccine candidates failed at earlier stages in trials.
New approaches, such as nucleic acid (DNA or mRNA) vaccines, and engineered replication-deficient viruses, may offer better prospects by soliciting a broader and longer-lasting immune response. A list of current candidates is shown below (11).
VZV Vaccines
Note: this section originally appeared in my post on VZV, I’m copying it here for easier reading.
Currently there are two FDA-approved vaccines for VZV (I’m counting different formulations of the same vaccine as one vaccine.)
The first to be developed was a live-attenuated strain, produced by serial passage of a wild-type virus in cell culture during the 1970s. The process of serial passage resulted in the virus undergoing genetic drift (mutations) that reduced its fitness to infect humans rather than cell lines. The “vaccine virus” is actually a population of mutated viruses, with 235 – 336 different mutations identified depending on the batch (15).
This is a very old-school method of making attenuated viruses, but in this case it was successful. The live-attenuated VZV vaccine is highly effective in preventing chickenpox after two doses, and breakthrough cases that do occur are usually mild. Interestingly, the vaccine is also effective for post-exposure prophylaxis, if given within 3 days of exposure. A different formulation of the vaccine (Zostavax) is approved to prevent shingles by boosting immunity in older adults.
Since the live-attenuated vaccine is a live virus, it still replicates within the host and establishes latency. This is actually a good thing since it ensures persistent protection. However, it can sometimes cause disease in immunocompromised people, and even can be transmitted between individuals (although it is much less infectious than wild-type). In rare cases, the vaccine virus can even mutate back to a more virulent form, although this is still not as bad as the wild-type virus (15). This is because the mutations that occurred during serial passage did not actually delete the viral genes, just inactivate them, leaving open the possibility for mutations to restore their function.
In order to allow the immunization of elderly adults with weakened immune systems who may not tolerate the live-attenuated vaccine, a recombinant protein vaccine (Shingrix) was developed by GlaxoSmithKline. This vaccine is a mixture of a truncated form of VZV gE and several adjuvants, including a saponin and lipopolysaccharide, which activate the immune system. It has been shown to be highly effective (94 – 99%) in preventing shingles over a 3-year follow-up period (16). It is unclear whether it would also be effective in preventing primary VZV infection.
The successes of VZV vaccines have shown that it is possible to vaccinate against an alphaherpesvirus. However, many of the same strategies for VZV vaccines have already been tested against other herpesviruses, with disappointing results. Herpesvirus vaccines aren’t impossible (at least for alphaherpesviruses), but some innovation will be needed.
EBV Vaccines
EBV vaccines are currently in development for the prevention of infection, as well as for the treatment of EBV-positive cancers. So far, all vaccines tested in humans have been protein vaccines against gp350. These have shown moderate protection against symptomatic mononucleosis, but not against EBV infection (17, 18). Improved protein vaccines containing a broader variety of glycoproteins are in development.
Moderna also has a mRNA vaccine candidate, expected to start phase I trials soon (as of late 2021).
In general, developing an EBV vaccine faces many challenges. As mentioned in my original EBV post, even the strong anti-EBV immune response in EBV-infected individuals is inadequate at preventing superinfection by a new strain. Furthermore, since the severity of primary EBV infection increases with age, an EBV vaccine that provides imperfect or short-lived protection may do more harm than good, by delaying infection to an age where it is more severe.
CMV Vaccines
Due to the tremendous burden of disease caused by CMV, developing an effective vaccine would be a great benefit to humanity. Indeed, the U.S. National Academy of Medicine has placed it as its top vaccine priority for over two decades (19). A 2019 review article by Stanley Plotkin (long may he live) and Suresh Boppana provides an excellent overview of past and current approaches to CMV vaccine development (20). I recommend reading this paper for yourself, but I will summarize a few key points here:
Multiple vaccine types (live-attenuated, conditional replication, protein subunit) are in development.
Prevention of CMV infection may be out of reach due to its powerful immune evasion, but some vaccine candidates have demonstrated protection from disease.
Even an imperfect vaccine may be useful if it prevents transmission from an infected pregnant woman to her fetus.
As of late 2021, Moderna’s anti-CMV mRNA vaccine candidate is now in phase III trials and recruiting participants. The estimated trial completion date is 2025. If you live near one of the trial centers, I highly recommend enrolling.
4. Human Germline Engineering
CRISPR/Cas systems are bacterial immune systems that fight viruses. What if we give humans those immune systems? Even a rather crudely developed proof-of-concept worked well against CMV in cell culture (21), and there is much room for optimization and improvement in gRNA target sequence, as well as which Cas protein to use (Cas9 is probably not the best).
In any case, the CRISPR system would have to be present in all the cells the virus could potentially infect, which means that unless some 100% effective delivery system is invented, it would have to be introduced into the human germline.
Pros:
highly effective
will eliminate latent infection
zero cost after initial setup
Cons:
not adaptive (need many gRNAs to prevent mutational escape)
there may be off-target cutting
currently illegal to do human germline editing
Still, this technology has the potential to provide complete protection against all known herpesviruses, and other viruses too. Even RNA viruses can be targeted with Cas13 (22). Overall, I consider this to be highly promising.
5. Conclusions
Hopefully, human germline editing will solve all our virus problems. Until then, we have antiviral drugs and vaccines. The drugs have side effects and the vaccines mostly don’t work, so there’s a large need to develop better ones. Fortunately, there are ongoing trials in this area; I especially encourage you to sign up for Moderna’s CMV vaccine trial, if you’re eligible.
6. References
1. A. J. Wagstaff, D. Faulds, K. L. Goa, Aciclovir: A Reappraisal of its Antiviral Activity, Pharmacokinetic Properties and Therapeutic Efficacy. Drugs. 47, 153–205 (1994).
2. M. R. Boyd, S. Safrin, E. R. Kern, Penciclovir: a review of its spectrum of activity, selectivity, and cross-resistance pattern. Antivir Chem Chemother. 4, 3–11 (1993).
3. F. M. Marty, M. Boeckh, Maribavir and human cytomegalovirus-what happened in the clinical trials and why might the drug have failed? Curr Opin Virol. 1, 555–562 (2011).
4. F. M. Marty, P. Ljungman, R. F. Chemaly, J. Maertens, S. S. Dadwal, R. F. Duarte, S. Haider, A. J. Ullmann, Y. Katayama, J. Brown, K. M. Mullane, M. Boeckh, E. A. Blumberg, H. Einsele, D. R. Snydman, Y. Kanda, M. J. DiNubile, V. L. Teal, H. Wan, Y. Murata, N. A. Kartsonis, R. Y. Leavitt, C. Badshah, Letermovir Prophylaxis for Cytomegalovirus in Hematopoietic-Cell Transplantation. N Engl J Med. 377, 2433–2444 (2017).
5. T. E. Messick, G. R. Smith, S. S. Soldan, M. E. McDonnell, J. S. Deakyne, K. A. Malecka, L. Tolvinski, A. P. J. van den Heuvel, B.-W. Gu, J. A. Cassel, D. H. Tran, B. R. Wassermann, Y. Zhang, V. Velvadapu, E. R. Zartler, P. Busson, A. B. Reitz, P. M. Lieberman, Structure-based design of small-molecule inhibitors of EBNA1 DNA binding blocks Epstein-Barr virus latent infection and tumor growth. Sci. Transl. Med. 11, eaau5612 (2019).
6. J. Maertens, A. C. Logan, J. Jang, G. Long, J.-L. Tang, W. Y. K. Hwang, L. P. Koh, R. Chemaly, A. Gerbitz, J. Winkler, S.-P. Yeh, J. Hiemenz, S. Christoph, D.-G. Lee, P.-N. Wang, E. Holler, S. Mielke, L. Akard, A. Yeo, S. Ramachandra, K. Smith, P. Pertel, F. Segal, Phase 2 Study of Anti-Human Cytomegalovirus Monoclonal Antibodies for Prophylaxis in Hematopoietic Cell Transplantation. Antimicrob Agents Chemother. 64 (2020), doi:10.1128/AAC.02467-19.
7. E. B. Struble, H. Murata, T. Komatsu, D. Scott, Immune Prophylaxis and Therapy for Human Cytomegalovirus Infection. IJMS. 22, 8728 (2021).
8. K. Poppiti, A. Lin, N. G. Adel, P. Hilden, H. Castro-Malaspina, Outcomes of Rituximab for EBV Viremia/Post-Transplant Lymphoproliferative Disease in CD34+ Selected Allogeneic Hematopoietic Stem Cell Transplantation. Blood. 128, 4623–4623 (2016).
9. Q.-Y. Zhu, S. Shan, J. Yu, S.-Y. Peng, C. Sun, Y. Zuo, L.-Y. Zhong, S.-M. Yan, X. Zhang, Z. Yang, Y.-J. Peng, X. Shi, S.-M. Cao, X. Wang, M.-S. Zeng, L. Zhang, A potent and protective human neutralizing antibody targeting a novel vulnerable site of Epstein-Barr virus. Nat Commun. 12, 6624 (2021).
10. A. F. Read, S. J. Baigent, C. Powers, L. B. Kgosana, L. Blackwell, L. P. Smith, D. A. Kennedy, S. W. Walkden-Brown, V. K. Nair, Imperfect Vaccination Can Enhance the Transmission of Highly Virulent Pathogens. PLoS Biol. 13, e1002198 (2015).
11. R. Krishnan, P. M. Stuart, Developments in Vaccination for Herpes Simplex Virus. Front. Microbiol. 12, 798927 (2021).
12. L. Corey, A. G. Langenberg, R. Ashley, R. E. Sekulovich, A. E. Izu, J. M. Douglas, H. H. Handsfield, T. Warren, L. Marr, S. Tyring, R. DiCarlo, A. A. Adimora, P. Leone, C. L. Dekker, R. L. Burke, W. P. Leong, S. E. Straus, Recombinant glycoprotein vaccine for the prevention of genital HSV-2 infection: two randomized controlled trials. Chiron HSV Vaccine Study Group. JAMA. 282, 331–340 (1999).
13. L. R. Stanberry, S. L. Spruance, A. L. Cunningham, D. I. Bernstein, A. Mindel, S. Sacks, S. Tyring, F. Y. Aoki, M. Slaoui, M. Denis, P. Vandepapeliere, G. Dubin, Glycoprotein-D–Adjuvant Vaccine to Prevent Genital Herpes. N Engl J Med. 347, 1652–1661 (2002).
14. R. B. Belshe, P. A. Leone, D. I. Bernstein, A. Wald, M. J. Levin, J. T. Stapleton, I. Gorfinkel, R. L. A. Morrow, M. G. Ewell, A. Stokes-Riner, G. Dubin, T. C. Heineman, J. M. Schulte, C. D. Deal, Efficacy Results of a Trial of a Herpes Simplex Vaccine. N Engl J Med. 366, 34–43 (2012).
15. D. P. Depledge, S. Kundu, N. J. Jensen, E. R. Gray, M. Jones, S. Steinberg, A. Gershon, P. R. Kinchington, D. S. Schmid, F. Balloux, R. A. Nichols, J. Breuer, Deep Sequencing of Viral Genomes Provides Insight into the Evolution and Pathogenesis of Varicella Zoster Virus and Its Vaccine in Humans. Molecular Biology and Evolution. 31, 397–409 (2014).
16. H. Lal, A. L. Cunningham, O. Godeaux, R. Chlibek, J. Diez-Domingo, S.-J. Hwang, M. J. Levin, J. E. McElhaney, A. Poder, J. Puig-Barberà, T. Vesikari, D. Watanabe, L. Weckx, T. Zahaf, T. C. Heineman, Efficacy of an Adjuvanted Herpes Zoster Subunit Vaccine in Older Adults. N Engl J Med. 372, 2087–2096 (2015).
17. E. M. Sokal, K. Hoppenbrouwers, C. Vandermeulen, M. Moutschen, P. Léonard, A. Moreels, M. Haumont, A. Bollen, F. Smets, M. Denis, Recombinant gp350 Vaccine for Infectious Mononucleosis: A Phase 2, Randomized, Double‐Blind, Placebo‐Controlled Trial to Evaluate the Safety, Immunogenicity, and Efficacy of an Epstein‐Barr Virus Vaccine in Healthy Young Adults. J INFECT DIS. 196, 1749–1753 (2007).
18. V. Jean-Pierre, J. Lupo, M. Buisson, P. Morand, R. Germi, Main Targets of Interest for the Development of a Prophylactic or Therapeutic Epstein-Barr Virus Vaccine. Front. Microbiol. 12, 701611 (2021).
19. J. F. Modlin, A. M. Arvin, P. Fast, M. Myers, S. Plotkin, R. Rabinovich, Vaccine Development to Prevent Cytomegalovirus Disease: Report from the National Vaccine Advisory Committee. Clinical Infectious Diseases. 39, 233–239 (2004).
20. S. A. Plotkin, S. B. Boppana, Vaccination against the human cytomegalovirus. Vaccine. 37, 7437–7442 (2019).
21. J. Gergen, F. Coulon, A. Creneguy, N. Elain-Duret, A. Gutierrez, O. Pinkenburg, E. Verhoeyen, I. Anegon, T. H. Nguyen, F. A. Halary, F. Haspot, Multiplex CRISPR/Cas9 system impairs HCMV replication by excising an essential viral gene. PLoS ONE. 13, e0192602 (2018).
22. T. R. Abbott, G. Dhamdhere, Y. Liu, X. Lin, L. Goudy, L. Zeng, A. Chemparathy, S. Chmura, N. S. Heaton, R. Debs, T. Pande, D. Endy, M. F. La Russa, D. B. Lewis, L. S. Qi, Development of CRISPR as an Antiviral Strategy to Combat SARS-CoV-2 and Influenza. Cell. 181, 865-876.e12 (2020).
To encourage vaccine trial participation and to lower the barrier, a link to the trial would be helpful at the end of the post. https://clinicaltrials.gov/ct2/show/NCT05085366
It might also make sense to outline the possible benefits of anybody participating - if you're in the active group, you will likely have (limited?) protection against CMV. Possibly avoiding some of the nasty long-term ramifications of CMV.
Love this series, thanks so much for writing it, your love of learning really comes across.