Cytomegalovirus: The worst herpesvirus
Part 5 of “The human herpesviruses: much more than you wanted to know”
The worst herpesvirus is one you’ve probably never heard of.
Human herpesvirus 5 (CMV) infects the majority of humanity, and on a global scale, is about as bad as HIV and malaria. Here's what you can do to avoid infecting (and superinfecting) yourself and your children.
(This cytomegaloblogpost is Part 5 of my herpesvirus series. Previous parts are here.)
0. Actionable advice
The only way to prevent CMV infection is to avoid exposure to the bodily fluids of others. Even if you’re already CMV-positive, you can still be superinfected.
Avoiding CMV is especially important for pregnant women, since infection during pregnancy causes developmental defects in the fetus.
If you are CMV-positive and planning to breastfeed, you should pasteurize your breastmilk (or at least freeze it, which is partially effective).
Young children often shed large amounts of CMV. While putting children in daycare is unavoidable for some families, you should know that it comes with a substantial risk of CMV infection.
1. Introduction
In my previous post, I wrote that Epstein-Barr virus was a strong contender for the worst human herpesvirus.
I was wrong.
Cytomegalovirus (CMV) is worse, and it’s not even close. Given that only a small fraction of people have ever heard of CMV (1), clearly more awareness is needed.
There are two reasons that CMV is terrible: first, it causes birth defects, and second, it accelerates aging. I’ll discuss both below. I’ve estimated the worldwide annual disability-adjusted life year (DALY) cost of CMV due to both factors (see sections 6, 8, and 9), and it’s probably somewhere between HIV and TB in terms of badness.
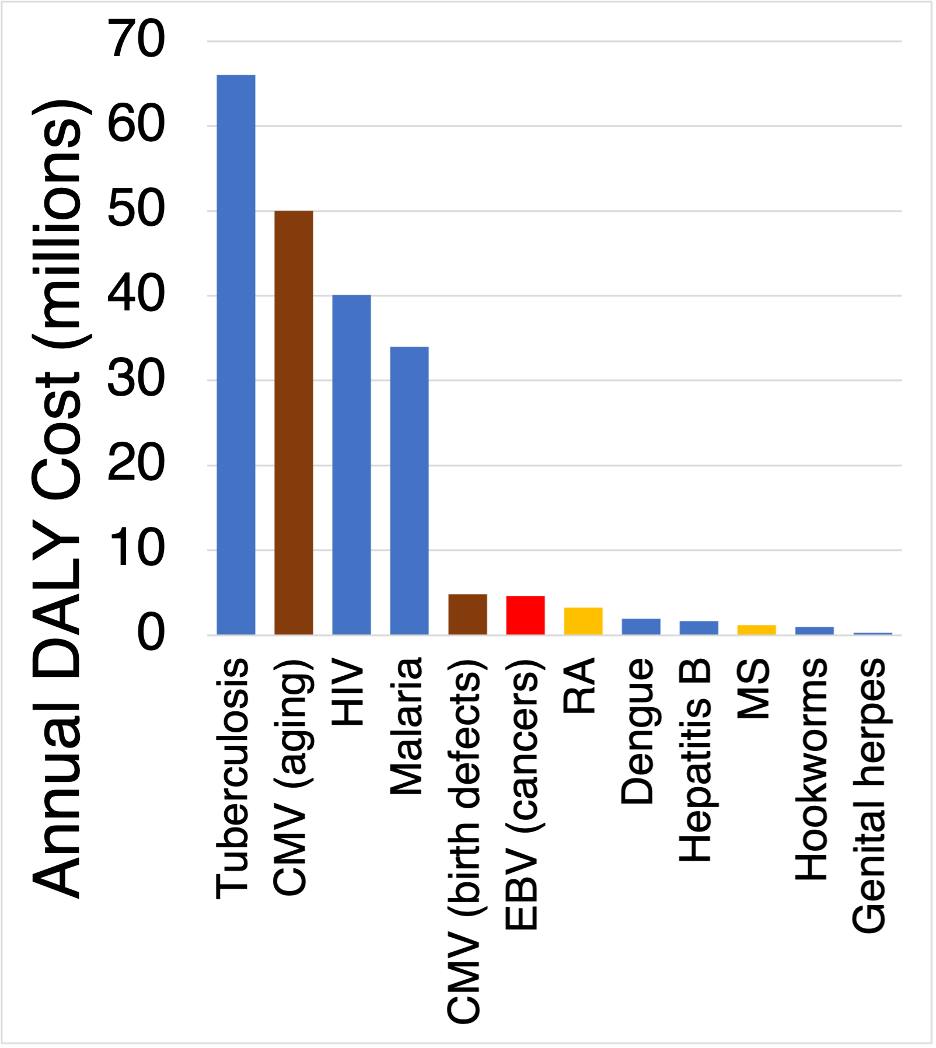
Given the huge cost of CMV, it should definitely be getting more attention than it currently is.
2. Epidemiology of CMV
CMV infects the majority of humanity, although its prevalence is not uniform within different populations (2). In developing countries, rates of CMV infection approach 100% and about 1 in 70 babies is born infected (3). In the United States and other high-income countries, the overall rate of CMV infection is about 50% and about 1 in 150 babies is born infected. The rate of infection increases steadily with age.
CMV easily spreads in daycares, since toddlers aren’t great about keeping their saliva to themselves. A meta-analysis found that on average, 23% of children in daycares were actively shedding CMV in saliva or urine, compared to 12% of children of similar ages who were not in daycares (4). A study in one daycare showed that CMV-negative children had a risk of infection of 12.6% per year (5).
Rates of CMV infection are higher in people with more sexual partners (6). This makes sense because sexual contact spreads CMV, either via saliva or genital secretions (the two modes of transmission are hard to distinguish). Unfortunately, I couldn’t find any data on the actual risk of transmission per sexual encounter.
3. CMV Transmission
The infectious dose of CMV is quite small. In a vaccine challenge trial, a dose of 10 PFU (equivalent to 10 viable virions) was sufficient to infect all CMV-seronegative volunteers (7).
*Record scratch*
There was a CMV challenge trial? CMV establishes lifelong infection and has particular risks if transmitted to vulnerable individuals (pregnant women, infants, the immunosuppressed, etc.).
How would a challenge trial be in any way acceptable? I was shocked to read that Stanley Plotkin (one of my personal heroes) had conducted such as study in 1989. Then, I read a bit further, and thought, “Wow, that’s a genius choice of a subject population.”
The researchers recruited Catholic monks, who were about as low-risk as possible, and more importantly, were very unlikely to transmit CMV to others. Anyway, they found that 10 PFU, delivered subcutaneously, could infect seronegative individuals. They didn’t test lower doses, and it’s likely that a single virion is sufficient to cause infection. Superinfection of seropositive individuals was also possible at higher doses (100 – 1000 PFU). To put this in context, urine from CMV-positive children can contain up to 100,000 PFU/mL (5). I don’t know how the subcutaneous infectious dose compares to the infectious dose for other routes (e.g. oral).
In infected individuals, CMV is shed in every bodily fluid, including saliva, breast milk, genital secretions, and urine. There is a common myth that urine is sterile, but this is blatantly incorrect, given that it’s a major route of CMV transmission. CMV can also be transmitted via the placenta from an infected mother to her fetus. (More on this below.)
For lactating CMV-positive women, the risk of transmission via breastmilk can be eliminated by pasteurization or irradiation. Freezing, which is easier to perform, is partially effective, reducing the risk of transmission by about 75% (8).
CMV can also be transmitted by blood transfusion. Blood products are not generally screened for CMV, and a large fraction of donors are CMV-positive. The medical establishment doesn’t seem to care about this, and takes precautions only for immunocompromised patients. In these cases, blood to be transfused is first filtered to remove donor leukocytes. At first I was skeptical that this was actually effective at removing CMV, since virions are too small to be removed by this filtration. So, I asked Dr. Lakshmi Ganapathi, an infectious diseases specialist at Boston Children’s hospital who often treats immunocompromised children. She explained that CMV virions are present in the blood only during primary infection, and the risk of bloodborne transmission is almost entirely due to the transmission of latently infected leukocytes. This contrasts to other fluids such as saliva, urine, and breastmilk, which do have free virions. Therefore, filtration is highly effective in reducing the risk of transfusion-related CMV transmission (9), and is non-inferior to serological screening (which would not detect primary CMV infections anyway, due to the delay in antibody response).
4. How to tell if you’re infected
Edit 2021-10-18: Commenters on Reddit have pointed out that some blood banks test for CMV. The easiest way to get tested is probably to find one of these blood banks near you, and donate blood there.
Most CMV infections are asymptomatic, although some cases are accompanied by a mononucleosis-like syndrome. CMV testing is not routinely performed for healthy people (even for blood donors). There are some labs that do it, but you’ll have to pay out of pocket (a few hundred dollars).
I’m currently working on a method to try to detect CMV in whole genome sequence data (from saliva). If this is successful I’ll post about it. I’m not sure how sensitive this will be, though.
In any case, due to the possibility of superinfection or [REDACTED], you should try to avoid exposure to CMV regardless of your current status.
5. Virology of CMV
Even for a herpesvirus, CMV is remarkably complex. Its large genome size (240–250 kbp, nearly twice that of VZV) enables it to encode at least 751 different proteins (10), and for most of these, the function is still unknown. Although there’s still a lot of work to be done in understanding CMV, after decades of research the basics are now relatively well established.
CMV, also known as human herpesvirus 5, is a betaherpesvirus that infects a wide variety of cell types, including epithelial cells, endothelial cells, fibroblasts, kidney cells, neurons, hepatocytes, and lymphocytes. I expect this list is far from complete; this is just what’s known so far. The name “cytomegalovirus” comes from the fact that infected cells swell up with virions, making them larger.
CMV’s entry into target cells is mediated by several viral glycoproteins. Some of these (e.g. gB, gH, gL, and gO) are universally essential for entry, while others enhance entry into particular cell types (11). CMV can use many different cell surface molecules as receptors, and basically every human cell type expresses one or more of these targets. Within a host, CMV can also spread directly from cell to cell, without releasing viral particles that might be targeted by antibodies.
Like the other herpesviruses, CMV can either begin active replication or establish latency after entering a host cell. The pattern of gene expression during active infection proceeds in a well-defined sequence (12). The first CMV genes transcribed during active replication are known as the “immediate-early” (IE) genes, and their protein products activate various viral promoters, leading to expression of “early” genes. One of these is the CMV DNA polymerase, which mediates genome replication during active infection, and is the target of all known anti-CMV drugs. After replication is underway, “late” genes, encoding viral structural proteins, become expressed to allow the assembly of new virions, which assemble in the nucleus of the host cell and are transported via the Golgi apparatus to be released from the cell surface.
Alternatively, CMV can establish latency in the cells that it infects. The maintenance of latency is coordinated by several viral proteins and microRNAs, as well as host cell proteins (13). During latency, a few viral proteins are still expressed; these proteins can influence the behavior of latently infected immune cells and cause them to disseminate throughout the body. The viral genome is present as an episome, which is replicated by host DNA polymerase when the host cell divides.
The broad tropism of CMV, as well as its astonishingly powerful immune evasion, means that a substantial fraction of cells in otherwise healthy individuals may be latently infected. Leukocytes from CMV-positive blood donors harbor latent CMV at a rate of approximately 1 per 10^4 to 10^5 (14). This may seem small, but it’s roughly equivalent to one CMV-infected cell per milliliter of blood, and more in immune tissues (bone marrow, lymph nodes, etc.). I couldn’t find humans data for other tissues, but for mouse CMV, there can be up to 1 latent CMV genome per 40 lung cells (15).
CMV reactivation from latency is a complicated process, but one thing that is known to cause reactivation is lactation. CMV reactivates in the mammary gland during breastfeeding, which helps its chances of transmission from mother to child. Nearly all CMV-positive women will shed CMV in their milk (8). Interestingly, this reactivation is restricted to mammary tissues between weeks 1 – 12 postpartum. CMV also intermittently reactivates in the salivary gland and kidney, leading to shedding in urine and saliva.
After a millions-of-years-long winning streak, CMV is the undisputed champion of the immune evasion game, with many devious tricks to avoid detection (16). For example, CMV blocks detection of its own antigens by downregulating host MHC receptors. Normally, MHC receptors display viral antigens on the cell surface, but CMV makes them get stuck in the endoplasmic reticulum, and also targets them for degradation. CMV isn’t the only virus that does this, and understandably the lack of MHC receptors on the cell surface is a giant red flag for natural killer T cells to destroy potentially infected cells. However, CMV encodes its own decoy MHC proteins to fool the killer T’s and escape destruction.
In addition to the decoy MHC, CMV also expresses a mimic of interleukin 10, an immunosuppressive signaling molecule. Furthermore, CMV is known to interfere with the innate immune system, which senses “pathogen-associated molecular patterns” that are signals of infection (17). Besides disrupting multiple different sensor molecules, CMV also shuts off the interferon signaling pathway downstream of these sensors, which normally raises the alarm and tells the immune system that a cell is virally infected. This is just a brief overview of the most important immune evasion mechanisms, but there are many others as well.
CMV is so good at evading the immune system that it can “superinfect”, where an individual who is already latently infected develops a second primary infection. This is quite unusual for a virus. In the case of CMV, superinfection depends on its ability to evade the response of CD8+ T-cells (18). The possibility of superinfection is a discouraging sign for vaccine development, since it indicates that the normal immune response is inadequate to prevent CMV infection.
6. CMV and birth defects
CMV is the most common congenital viral infection, and approximately 1 in 150 infants in the United States is born with CMV.
Congenital CMV infection is the single largest non-genetic cause of birth defects, surpassing even fetal alcohol syndrome (19). Approximately 20% of congenital CMV cases are accompanied by moderate to severe developmental disabilities, including deafness and mental retardation. In severe cases it can cause fatal organ failure, which is particularly a risk for premature or otherwise immunocompromised infants. Even in “mild” cases of congenital CMV, subtle deficits may be present.
Paradoxically, women who are CMV-seropositive prior to pregnancy are 69% less likely to have a baby with congenital CMV (20). This is because maternal antibodies are partially effective in blocking CMV transmission to the fetus. The greatest risk is for a seronegative mother who develops a primary CMV infection during pregnancy. Still, this only accounts for 25% of congenital CMV cases, with the other 75% of cases being due to a mother’s latent infection (19).
Interestingly, it’s been hypothesized that romantic kissing evolved as a method to reduce congenital CMV by ensuring that the mother was infected prior to pregnancy, rather than during pregnancy (21). I don’t know if this has any evidence, but it seems plausible. Overall I would still recommend trying to avoid CMV exposure prior to pregancy.
CMV infections in infants, while not as high-risk as prenatal infections, can also cause long-term problems such as deafness. Infants born to seropositive mothers are at lower risk of severe disease due to transfer of maternal antibodies, but this protection fades after a few weeks.
On average, each case of congenital CMV results in the loss of ~3 DALYs (22). Given 140 million babies are born per year (as of 2021) (23), if the rate of congenital CMV is ~1% of births, this would result in an annual cost of 4.2 million DALYs. While this is large, the majority of CMV’s DALY burden comes from its effects on aging (see below).
7. CMV in vulnerable patients
CMV is a serious threat to immunocompromised individuals. Organ transplant patients, who are immunosuppressed to avoid transplant rejection, are often prescribed valganciclovir prophylaxis against CMV (24). The risk is greatest when an organ is transplanted from a CMV-positive donor to a negative recipient. CMV prophylaxis is generally continued for only 6 to 12 months after transplantation, since after this period, patients can transition to “maintenance immunosuppression” where their immune systems aren’t quite as suppressed. Furthermore, long-term use of valganciclovir can cause kidney and liver damage (which is especially a risk in kidney or liver transplant patients). Historically, anti-CMV antibodies were used as prophylaxis, but CMV’s immune evasion made these not very effective.
According to Dr. Ganapathi, the best method of preventing CMV disease in transplant recipients is still an area of active research, with some hospitals foregoing prophylaxis and instead doing weekly PCR testing, with valganciclovir treatment initiated if a high CMV load is detected.
Prior to the identification of HIV, CMV was suggested as a cause of AIDS, because the prevalence was much higher in gay men than in the general population (25), and also because AIDS patients with weakened immune systems often had extremely high viral loads of CMV (26). CMV, with its broad tropism, can cause a wide variety of problems in AIDS patients, such as hepatitis, colitis, encephalitis, and retinitis. Interestingly, CMV does actually cause immune dysfunction, although not as bad as AIDS.
8. CMV and immune dysfunction
CMV infection also harms otherwise healthy individuals, although this is more subtle. The human immune system struggles to control CMV, and over time this uses up an increasingly large fraction of its resources, especially T cells. “T-cell inflation”, as this process is called, means that roughly 30% of all CD8+ T cells in CMV-infected adults are specifically fighting CMV (27). This fraction generally increases with age.
T-cell inflation leaves fewer T cells available to combat other viral infections and cancers. For example, CMV-positive people are at higher risk of severe COVID-19 (although this was a correlational study and may be confounded by demographic factors) (28). Other research has found that CMV-infected elderly adults have reduced T-cell responses to influenza (29), and influenza vaccines cause less of a B-cell response in CMV-positive individuals (30). However, this is still controversial, as another study found the opposite effect, at least in young adults (31). CMV, although not oncogenic like EBV, is also associated with several kinds of cancers (32).
9. CMV and aging
Besides immunosenescence, inflammation caused by CMV infection is associated with aging in several tissues. Various studies found that CMV-positive individuals have an increased risk of type 2 diabetes, coronary artery disease, ischemic heart disease, and general frailty (33). CMV infection (as with other herpesvirus infections) is also associated with an increased risk of idiopathic pulmonary fibrosis (34). CMV is particularly associated with vascular disease, since it infects the lining of blood vessels and causes inflammation there.
Overall, CMV-positive individuals have an increased all-cause mortality. Unadjusted, the effect seems enormous, but this is largely due to the fact that older people are more likely to be CMV-positive. Even after adjusting for this and other confounders, the estimated effect is still a 1.19-fold increase in all-cause mortality (35), or a loss of approximately 1.1 years of life expectancy in the study population of adults of 25 years and older.
Given that CMV infects most of humanity, its overall cost in DALYs due to aging is likely quite high. A rough calculation places it at around 50 million DALYs annually (~50 million total deaths per year in CMV-positive people globally, each of which hastened by ~1 year). This should be adjusted downwards since not all deaths are aging-related, but also upwards since CMV may cause aging-related disability before death. Overall I think 50 million is a good estimate; my overall confidence interval is between 10 and 100 million. This range makes it worse than EBV by far, and around the same level as HIV. Again, this is just an estimate, but I expect it to be roughly correct.
10. CMV in biotechnology
Despite being generally terrible, CMV has provided a few benefits to biotechnology.
Sometimes scientists need an extremely strong promoter, one that tells cells, “Make as much of this mRNA as possible”. The CMV major immediate/early promoter, known simply as “the CMV promoter”, has often been used for this purpose. In CMV, this promoter drives the expression of IE1 and IE2 proteins, produced from a single mRNA by alternative splicing. These “immediate early” proteins are the first ones to be expressed upon viral infection, and activate the expression of the rest of the viral genes.
Historically, the CMV promoter was one of the earliest ones used for transgene expression in mammalian cells, with the promoter sequence first published in 1984 (36).
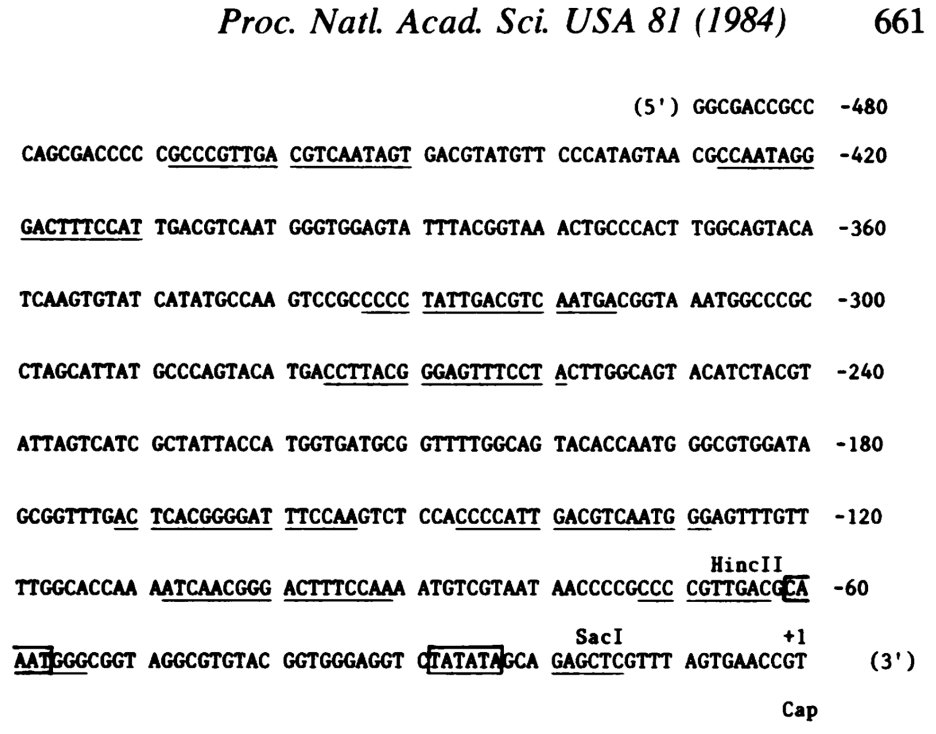
Since then, researchers have made several variations on the CMV promoter, and annoyingly, papers don’t often specify which one they used. Some will also contain the upstream enhancer region, but others lack it.
Although it provides very strong expression, the CMV promoter can be prone to silencing (where some cells methylate the promoter and shut it off, leading to uneven expression within a population). This makes sense, given the role of silencing in the establishment of latency. In my experience, other promoters such as EF1-alpha are more reliable. Still, the CMV promoter is still useful: combining other promoters with a “minimal CMV” promoter can result in better control over gene expression, while retaining the possibility of extremely high expression levels.
11. [REDACTED]
This section is available only on a need-to-know basis.
If you’re a biosecurity researcher or policymaker, and you want to see this section, you can contact me by email (metacelsus at protonmail.com).
12. Conclusions
Cytomegalovirus is by far the worst human herpesvirus. Although most infections are asymptomatic, CMV causes birth defects and accelerated aging, and is dangerous for people with weakened immune systems. CMV is shed in all bodily fluids; young children typically shed the highest amounts but shedding continues throughout life. Due to its excellent immune evasion, immunity provided by an existing CMV infection does not adequately protect against a new CMV infection, and transmission can be prevented only by avoiding exposure. Developing ways to prevent and treat CMV should be a high priority for society, especially due to [REDACTED].
13. Acknowledgments
I thank Dr. Lakshmi Ganapathi for information about CMV, organ transplants, and blood transfusions, and Alexey Guzey for feedback on a draft version of this post.
14. References
1. D. S. Ross, M. Victor, E. Sumartojo, M. J. Cannon, Women’s Knowledge of Congenital Cytomegalovirus: Results From the 2005 HealthStylesTM Survey. Journal of Women’s Health. 17, 849–858 (2008).
2. M. J. Cannon, D. S. Schmid, T. B. Hyde, Review of cytomegalovirus seroprevalence and demographic characteristics associated with infection: CMV seroprevalence. Rev. Med. Virol. 20, 202–213 (2010).
3. P. Ssentongo, C. Hehnly, P. Birungi, M. A. Roach, J. Spady, C. Fronterre, M. Wang, L. E. Murray-Kolb, L. Al-Shaar, V. M. Chinchilli, J. R. Broach, J. E. Ericson, S. J. Schiff, Congenital Cytomegalovirus Infection Burden and Epidemiologic Risk Factors in Countries With Universal Screening: A Systematic Review and Meta-analysis. JAMA Netw Open. 4, e2120736 (2021).
4. M. J. Cannon, T. B. Hyde, D. S. Schmid, Review of cytomegalovirus shedding in bodily fluids and relevance to congenital cytomegalovirus infection: Review of CMV shedding in bodily fluids. Rev. Med. Virol. 21, 240–255 (2011).
5. J. R. Murph, The Natural History of Acquired Cytomegalovirus Infection Among Children in Group Day Care. Arch Pediatr Adolesc Med. 142, 843 (1988).
6. T. M. Lanzieri, D. Kruszon-Moran, M. Gambhir, S. R. Bialek, Influence of parity and sexual history on cytomegalovirus seroprevalence among women aged 20-49 years in the USA. International Journal of Gynecology & Obstetrics. 135, 82–85 (2016).
7. S. A. Plotkin, S. E. Starr, H. M. Friedman, E. Gonczol, R. E. Weibel, Protective Effects of Towne Cytomegalovirus Vaccine Against Low-Passage Cytomegalovirus Administered as a Challenge. Journal of Infectious Diseases. 159, 860–865 (1989).
8. K. Hamprecht, J. Maschmann, G. Jahn, C. F. Poets, R. Goelz, Cytomegalovirus transmission to preterm infants during lactation. Journal of Clinical Virology. 41, 198–205 (2008).
9. D. Jebakumar, P. Bryant, W. Linz, Risk of cytomegalovirus transmission by blood products after solid organ transplantation. Baylor University Medical Center Proceedings. 32, 222–226 (2019).
10. N. Stern-Ginossar, B. Weisburd, A. Michalski, V. T. K. Le, M. Y. Hein, S.-X. Huang, M. Ma, B. Shen, S.-B. Qian, H. Hengel, M. Mann, N. T. Ingolia, J. S. Weissman, Decoding Human Cytomegalovirus. Science. 338, 1088–1093 (2012).
11. C. Nguyen, J. Kamil, Pathogen at the Gates: Human Cytomegalovirus Entry and Cell Tropism. Viruses. 10, 704 (2018).
12. M. P. Weekes, P. Tomasec, E. L. Huttlin, C. A. Fielding, D. Nusinow, R. J. Stanton, E. C. Y. Wang, R. Aicheler, I. Murrell, G. W. G. Wilkinson, P. J. Lehner, S. P. Gygi, Quantitative Temporal Viromics: An Approach to Investigate Host-Pathogen Interaction. Cell. 157, 1460–1472 (2014).
13. F. Goodrum, Human Cytomegalovirus Latency: Approaching the Gordian Knot. Annu. Rev. Virol. 3, 333–357 (2016).
14. B. Slobedman, E. S. Mocarski, Quantitative Analysis of Latent Human Cytomegalovirus. J Virol. 73, 4806–4812 (1999).
15. S. Kurz, H. P. Steffens, A. Mayer, J. R. Harris, M. J. Reddehase, Latency versus persistence or intermittent recurrences: evidence for a latent state of murine cytomegalovirus in the lungs. J Virol. 71, 2980–2987 (1997).
16. V. Noriega, V. Redmann, T. Gardner, D. Tortorella, Diverse immune evasion strategies by human cytomegalovirus. Immunol Res. 54, 140–151 (2012).
17. M. Biolatti, F. Gugliesi, V. Dell’Oste, S. Landolfo, Modulation of the innate immune response by human cytomegalovirus. Infection, Genetics and Evolution. 64, 105–114 (2018).
18. S. G. Hansen, C. J. Powers, R. Richards, A. B. Ventura, J. C. Ford, D. Siess, M. K. Axthelm, J. A. Nelson, M. A. Jarvis, L. J. Picker, K. Fruh, Evasion of CD8+ T Cells Is Critical for Superinfection by Cytomegalovirus. Science. 328, 102–106 (2010).
19. C. Wang, X. Zhang, S. Bialek, M. J. Cannon, Attribution of Congenital Cytomegalovirus Infection to Primary Versus Non-Primary Maternal Infection. Clinical Infectious Diseases. 52, e11–e13 (2011).
20. S. L. Bate, S. C. Dollard, M. J. Cannon, Cytomegalovirus Seroprevalence in the United States: The National Health and Nutrition Examination Surveys, 1988–2004. CLIN INFECT DIS. 50, 1439–1447 (2010).
21. C. A. Hendrie, G. Brewer, Kissing as an evolutionary adaptation to protect against Human Cytomegalovirus-like teratogenesis. Medical Hypotheses. 74, 222–224 (2010).
22. G. Rozhnova, M. E. Kretzschmar, F. van der Klis, D. van Baarle, M. Korndewal, A. C. Vossen, M. van Boven, Short- and long-term impact of vaccination against cytomegalovirus: a modeling study. BMC Med. 18, 174 (2020).
23. “Our World in Data: Births and deaths” (2021), (available at https://ourworldindata.org/births-and-deaths).
24. L. Ganapathi, J. Blumenthal, L. Alawdah, L. Lewis, J. Gilarde, S. Jones, C. Milliren, H. B. Kim, T. S. Sharma, Impact of standardized protocols for cytomegalovirus disease prevention in pediatric solid organ transplant recipients. Pediatr Transplant. 23 (2019), doi:10.1111/petr.13568.
25. W. L. Drew, L. Mintz, R. C. Miner, M. Sands, B. Ketterer, Prevalence of Cytomegalovirus Infection in Homosexual Men. Journal of Infectious Diseases. 143, 188–192 (1981).
26. Y. Becker, G. Darai, E.-S. Huang, Eds., Molecular Aspects of Human Cytomegalovirus Diseases (Springer Berlin Heidelberg, Berlin, Heidelberg, 1993; http://link.springer.com/10.1007/978-3-642-84850-6), vol. 2 of Frontiers of Virology.
27. P. Klenerman, A. Oxenius, T cell responses to cytomegalovirus. Nat Rev Immunol. 16, 367–377 (2016).
28. E. Shrock, E. Fujimura, T. Kula, R. T. Timms, I.-H. Lee, Y. Leng, M. L. Robinson, B. M. Sie, M. Z. Li, Y. Chen, J. Logue, A. Zuiani, D. McCulloch, F. J. N. Lelis, S. Henson, D. R. Monaco, M. Travers, S. Habibi, W. A. Clarke, P. Caturegli, O. Laeyendecker, A. Piechocka-Trocha, J. Z. Li, A. Khatri, H. Y. Chu, MGH COVID-19 Collection & Processing Team16, A.-C. Villani, K. Kays, M. B. Goldberg, N. Hacohen, M. R. Filbin, X. G. Yu, B. D. Walker, D. R. Wesemann, H. B. Larman, J. A. Lederer, S. J. Elledge, Viral epitope profiling of COVID-19 patients reveals cross-reactivity and correlates of severity. Science. 370, eabd4250 (2020).
29. E. Derhovanessian, A. B. Maier, K. Hähnel, J. E. McElhaney, E. P. Slagboom, G. Pawelec, Latent Infection with Cytomegalovirus Is Associated with Poor Memory CD4 Responses to Influenza A Core Proteins in the Elderly. J.I. 193, 3624–3631 (2014).
30. D. Frasca, A. Diaz, M. Romero, A. M. Landin, B. B. Blomberg, Cytomegalovirus (CMV) seropositivity decreases B cell responses to the influenza vaccine. Vaccine. 33, 1433–1439 (2015).
31. D. Furman, V. Jojic, S. Sharma, S. S. Shen-Orr, C. J. L. Angel, S. Onengut-Gumuscu, B. A. Kidd, H. T. Maecker, P. Concannon, C. L. Dekker, P. G. Thomas, M. M. Davis, Cytomegalovirus infection enhances the immune response to influenza. Sci. Transl. Med. 7, 281ra43-281ra43 (2015).
32. X. Luo, Q. Meng, M. Rao, Z. Liu, G. Paraschoudi, E. Dodoo, M. Maeurer, The impact of inflationary cytomegalovirus‐specific memory T cells on anti‐tumour immune responses in patients with cancer. Immunology. 155, 294–308 (2018).
33. A. E. Aiello, Y.-L. Chiu, D. Frasca, How does cytomegalovirus factor into diseases of aging and vaccine responses, and by what mechanisms? GeroScience. 39, 261–271 (2017).
34. G. Sheng, P. Chen, Y. Wei, H. Yue, J. Chu, J. Zhao, Y. Wang, W. Zhang, H.-L. Zhang, Viral Infection Increases the Risk of Idiopathic Pulmonary Fibrosis. Chest. 157, 1175–1187 (2020).
35. A. M. Simanek, J. B. Dowd, G. Pawelec, D. Melzer, A. Dutta, A. E. Aiello, Seropositivity to Cytomegalovirus, Inflammation, All-Cause and Cardiovascular Disease-Related Mortality in the United States. PLoS ONE. 6, e16103 (2011).
36. D. R. Thomsen, R. M. Stenberg, W. F. Goins, M. F. Stinski, Promoter-regulatory region of the major immediate early gene of human cytomegalovirus. Proceedings of the National Academy of Sciences. 81, 659–663 (1984).
I must admit that I've been quite shocked by your blog posts on herpes viruses. I was unaware of how easy transmission was, and I was treating them more like an "avoidable" disease (ala HIV) rather than one you get through just leading a "normal" life (ala certain HPVs).
I am curious though, if it's something you're even willing to discuss:
1. How good do you think the evidence of prevalence in young demographics is? As in, for all herpesviruses, it seems to increase almost linearly with age... which makes little sense prima-facia. If you haven't gotten it by the age of 30-40 (when most people marry and stabilize their friend groups, thus severely reducing the number of infection vectors), it seems reasonable to assume the chances of you getting it are low. Would you entertain a hypothesis where most (60-80%) of people are carriers of most HVs since birth or a young age, but only end up shedding the virus in detectable amounts, or getting an infection serious enough to get an immune-adaptive response at an older age?
2. If one was convinced to try and test themselves for various HVs (in order to assess risk to sexual partners and close friends/family. How indicative (if at all) would you think IgG levels are? And what methods of measuring IgG have the specificity to identify a latent infection? (I tried looking this up and it seems impossible from a layman-y perspective to get anything close to an obvious or consensus answer)
3. (maybe a bit personal) do you actually consider the impact of HVs to be enough to warrant lifestyle changes ? (i.e. not engaging with untested sexual partners, severely reducing the amount of food and drink that you share, prophylaxis after being in contact with possible infection vectors, etc). Does it seem reasonable to assume that anti-viral therapies would progress enough in the case of these viruses to adopt a "don't care" approach?
Ultimately , if you don't really have answers or feel comfortable giving them, no worries. I get that the level you're communicating this too is probably "medical researcher and policy maker" and I'm asking dumb clinical~ish questions that aren't your job. But I think you're answers would be interesting.
Also... have you considered doing HPV next?
This herpes virus series is very interesting. I have given birth to five children and I have heard a lot about toxoplasmosis and listeria and the smoked salmon and cheese and cats to avoid, but I never heard of CMV and the kisses to avoid.
Calling women of childbearing age "vulnerable people" implies a challenge to hook-up culture. It is not necessarily wrong, just very radical in current Western society.
I have to admit this new knowledge makes me a bit nervous when I take care of my two months old baby: can I sing to him at a close range? Or will those droplets of saliva we now all know are there when people sing give him cytolomegavirus? Age-old human social behaviour gets questioned with this kind of new knowledge.